For billions of years, our planet was nothing like Earth. It was Earth, of course. But if you looked at it from space, it kind of resembled a giant orange jawbreaker.
Most people don’t realize that Earth’s atmosphere wasn’t always the one we have right now. Its first atmosphere presumably had a lot of hydrogen gas, which was very quickly (relative to the planet’s 4.5-billion-year history) stripped away by solar wind. Earth regenerated a new atmosphere of primarily carbon dioxide, and maybe some water vapor and nitrogen, but no oxygen.
Yet there was life on the planet — anaerobic organisms to which oxygen was poisonous. Eventually, though, the cyanobacteria present in huge numbers on Earth’s surface began to produce oxygen as a byproduct of photosynthesis, and it built up in the atmosphere, causing a mass extinction. Life shifted into a different phase. Fueled by that oxygen, it evolved, became more complex. Advanced life on Earth, the air that we breathe, we owe to cyanobacteria from more than 2.5 billion years ago.
Jacob Bean, an astronomer and astrophysicist at the University of Chicago, is explaining all this to me in his fifth-floor office in the William Eckhardt Research Center, a gleaming glass building at 56th and Ellis. He speaks with a round and genial Southern accent that sounds like Matt Damon playing a farmer. Standing 5-foot-9, Bean, 43, has a strong jaw, a shaved head, and the posture of a cactus. Wearing a cream-colored sweater, dark jeans, and lime-aqua Hoka sneakers, he looks like a personal trainer, not someone who cocreated a pioneering astronomical instrument called MAROON-X, a much-flashier name than what it stands for: “M dwarf Advanced Radial velocity Observer Of Neighboring eXoplanets.”
For now, ignore what any of that means except for the last word. “Exoplanet” is short for “extrasolar planet,” denoting any planet outside our solar system — that is, not orbiting our sun. Astronomers estimate there are 100 billion stars in our galaxy alone, nearly all orbited by at least one planet, and 100 billion galaxies in the known universe. That’s a lot of planets! And endless avenues of inquiry for Bean, whose specialty is exoplanets. “It’s called job security,” Bean says.
For a long time, exoplanet science was a fringe sector of astrophysics. When Bean was a graduate student in the mid-2000s, virtually no one wanted to concentrate in it. Since then, however, aspiring astrophysicists have come to realize that exoplanets are the key to understanding not just the nature of planets but the evolution of Earth’s atmosphere, helping us fill in the gaps of our knowledge about our planet’s past and even its future. Today, Bean surmises that exoplanet specialists make up roughly 25 percent of the field. And within that community, he’s on the forefront. “He’s one of the world leaders in the field, without question,” says Sara Seager, an astrophysicist at the Massachusetts Institute of Technology.
In addition to running the university’s Bean Exoplanet Group, a nine-person research team, Bean coleads the Early Science Release Program on the James Webb Space Telescope, the trailblazing, paradigm-shifting instrument that launched in late 2021. That means he’s one of some 300 scientists who get the first look at data generated by the telescope, which he analyzes for atmospheric characterization of exoplanets.
It’s a good position to be in, because the telescope promises to revolutionize our understanding of these mysterious worlds. Astronomers have identified more than 5,000 exoplanets, but they don’t know all that much about them. With Webb, Bean and his colleagues will be able to observe these far-flung planets in greater detail than ever, allowing them to uncover new truths about how planets, including our own, form and evolve — and someday, perhaps, to discover other habitable worlds beyond our solar system. Maybe even another planet like Earth.
“The technology that we have has opened this window to the universe that we didn’t have before,” Bean says. “There’s a whole lot of questions that I think are really interesting that are answerable.” But he isn’t getting too far ahead of himself. “I’m living in the moment, and the moment is the planets that we’re able to study right now.”
That may be true. But because there’s still so much to learn, there’s also tremendous opportunity to make the kinds of discoveries that land scientists not just in textbooks but in the history books. And the biggest question for those who study exoplanets is perhaps the biggest question there is for humankind: Is anybody else out there?
Edward “Rocky” Kolb, who was the chair of the astronomy department at the U. of C., hired Bean in 2011. He had many reasons for selecting him, but Kolb makes sure to single out one in particular: “He had a goal in mind.”
And what was that goal?
“For people in the field, the thing that they really want to do, the big prize, the ring that you grab, how you go to Stockholm, is to discover life someplace else in the universe,” Kolb shouts, waving his hands in the air in an outward arc. “I think it will be done in our lifetime. And it’s going to happen once in human history. Jacob wants to do it. There’ll be a lot of people who play a role, but somebody’s going to do it.”
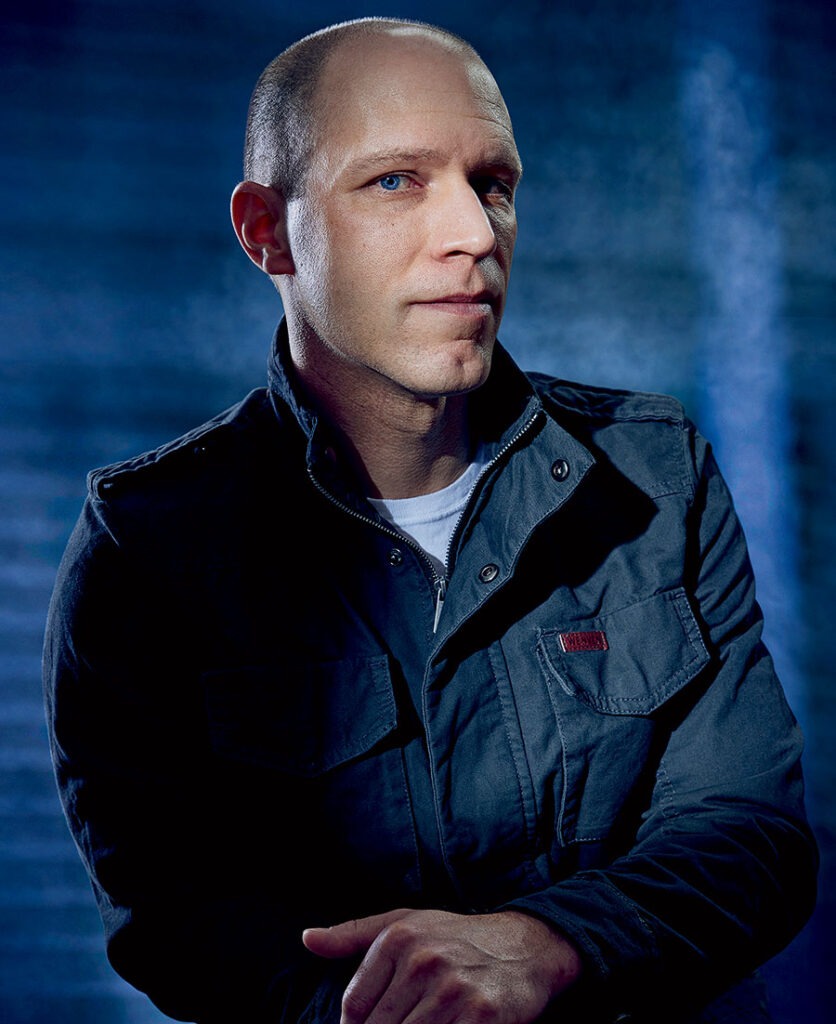
If Bean ends up discovering life elsewhere in the universe, I can’t imagine a more mundane place to do it from than his office. Even though he spends a lot of time sitting at his computer, there isn’t a stray piece of paper or a loose pen anywhere. The room feels less like the overstuffed library of a science nerd and more like the antiseptic domicile of a serial killer. To break the ice, I ask Bean if he was into The X-Files when he was younger. He just responds, “No,” without laughing. (This happened after he announced, unprompted, “I’m after the truth.”) “I’m a boring guy,” he says falsely.
He has a noticeable scar above his left eye, which he won’t talk about. “It’s not interesting,” he says.
Why not?
“Because it’s not interesting,” he says, twice, sternly.
However the mark originated, Bean asserts that it has nothing to do with rock climbing, a sport he pursues with near-monastic devotion. He climbs three days a week, which explains why he’s in incredible shape. When I ask what he loves about Chicago, he says, “It has an amazing number of climbing gyms.”
It’s a fitting hobby, because Bean is a flinty guy. “When I talk to people about Jacob or when they tell me about him, some people describe being kind of intimidated by meeting him, because he’s very straightforward and intense,” says Andreas Seifahrt, his longtime colleague. “That kind of approach, rough edges, there’s not a lot of social niceties.”
Bean rarely makes eye contact. And if he ever gets excited — by which I mean his voice rises a fraction of an octave — it’s invariably when he’s discussing science.
A simple question about astrophysics typically yields a 10-minute answer, a conversational map of his brain bouncing around among his joys, frustrations, and formidable knowledge of the discipline. His responses often end with a series of rhetorical questions, such as the ones that followed my query about the possibility of detecting a planet like Earth: “What’s the star like? What’s the high-energy output from the star now? What was it in the past? How would that interact with an atmosphere that probably formed? Can we say anything about the composition of that planet — and thus what kind of atmosphere it outcasts? What’s the chemistry in the atmosphere right now? Does that have any telltale clues about how lighter gases were lost over time?”
But the most animated I ever see Bean is when he’s talking about the James Webb Space Telescope.
Do you remember when NASA released the first images captured by the Webb telescope on July 12, 2022? I do. It felt like only one word could describe the pictures that appeared all over the news: Whoa.
Look no farther than — actually, scratch that. Look very far: at the Carina Nebula, a star-forming region in the Milky Way that’s about 7,500 light-years from Earth. Images of it already existed before Webb, taken by the Hubble Space Telescope. They are undeniably gorgeous, emanating the oceanic blue of a Chagall stained-glass window. But the Webb images are positively mind-blowing: cliffs of caramel clouds covered by a mist of Egyptian blue dappled with diamond twinkles, a nighttime backdrop in a cyborg-crafted psychedelic Disney cartoon.
The Webb images of exoplanets, however, aren’t especially ornate or spectacular — in fact, they’re pretty unimpressive. A rendering of WASP-39b, a Jupiter-like planet about 700 light-years from Earth, shows a sliver of a gaseous purple planet lit by a distant star. OK, kind of cool. But it lacks the stunning detail of Webb’s first transmissions. It looks a little like the cover of a bad dubstep album.
But that doesn’t make what’s pictured any less wondrous. Actually, it represents a groundbreaking discovery: In August 2022, a team co-led by Bean definitively detected carbon dioxide on the planet. It was a finding with enormous significance, because carbon dioxide had never before been conclusively found in an exoplanet’s atmosphere. And since carbon dioxide is a gas made up of one carbon atom bonded to two oxygen atoms, it meant the atmosphere contained oxygen. So why is that a big deal? After all, oxygen is the third-most-abundant element in the universe, right? Well, on Earth we have both carbon dioxide and molecular oxygen, or O2 (a molecule composed of two oxygen atoms bound together). The presence of oxygen doesn’t guarantee there’s life, but molecular oxygen might. “That’s the stuff we breathe produced by plant life on Earth, and we think that’s the smoking-gun evidence for life on a planet,” Bean says.
That’s not to say Bean and his colleagues can assert that life has ever existed on WASP-39b, or even that it has O2. But with each step in pinpointing chemical compounds that are the same as the ones we have on Earth, the nearer scientists get to determining if life has ever existed on those planets.
When I ask Bean how many discoveries like this are happening right now because of Webb, he shoots back, “I’ve got so much to say about that.” And he does. He begins by addressing something many exoplanet researchers have noted, which is that Webb’s performance has exceeded even their highest expectations. Bean references the throughput, or the amount of light that a telescope’s sensors can register. Earlier telescopes like Hubble had less throughput, so they could provide astronomers only limited data about a planet’s atmosphere.
The presence of oxygen doesn’t guarantee there’s life on another planet, but molecular oxygen might. “That’s the stuff we breathe produced by plant life on Earth, and we think that’s the smoking-gun evidence,” Bean says.
To explain the shortcomings of working with such data, Bean uses the parable of the blind men and the elephant. “It was like you’d see the toe, and scientists would infer, ‘It’s got a trunk, it’s gray.’ But still, all I’m seeing is a toe. I don’t know how big the elephant is. I can’t even say if the rest of it’s gray.” With Webb, you can now see the whole elephant.
Seeing the whole elephant introduced a new problem, though Bean is quick to say it’s a good one. After the WASP-39b breakthrough, Bean and his team later spotted carbon dioxide in other giant exoplanets similar to Jupiter. But since the amount of carbon dioxide is so different between these planets, it’s probable that they didn’t all form the same way, upending what astrophysicists believed they knew about them. “We’re struggling to separate out plausible scenarios,” Bean says. “These planets are not as homogeneous as we thought they were. And maybe we should have known better. The complexity is higher than what we anticipated, and that adds additional uncertainty.” In other words, with each new discovery, astrophysicists somehow get both closer to and further away from truly understanding how planets form and evolve.
A telescopic transmission comes back as pure data, incomprehensible to anyone outside the field . (It resembles what you’d see on an EKG monitor or a chart drawn by an old computer program — which is then mapped onto a graph before it’s handed over to artists for renderings.) The Webb data on exoplanets initially produced a flood of big revelations, but now comes the slow and painstaking process of sifting through the nuances and statistical imperfections, which may shift our knowledge of planets yet again.
Just as Earth wasn’t always the planet it is now, the exoplanets we’re studying weren’t always the way they are when they’re captured by a telescope. When something like Webb transmits data from a star, it’s not recording the present moment. As Bean reminds me, we’re seeing galaxies from the very beginning of the universe; Webb’s First Deep Field — the first image the telescope captured and the deepest ever view of the cosmos — is an image of galaxy cluster SMACS 0723 as it appeared 4.6 billion years ago, because of the time it takes for light to travel. By and large, astronomy is a snapshot of an object at one moment in its vast history. Even though Webb is extraordinarily powerful, it’s rare to see a planet change during observation.
We’re only beginning to scratch the surface. From Kepler, a space telescope launched in 2009 as part of NASA’s first planet-hunting initiative, we learned that sub-Neptune-size planets, which are larger than Earth but smaller than Neptune (whose radius is 3.88 times that of Earth), are the most common in the galaxy. Roughly half of all sun-like stars have sub-Neptune-size planets close to them. No one knows why. We don’t even know all that much about our own solar system’s Neptune.
Identifying more planets is still a significant chunk of exoplanet science. Of the 5,000 planets that astrophysicists can currently confirm, only a few hundred are feasible for atmospheric characterization, at least right now. And remember: There are 100 billion stars in our galaxy alone, to say nothing of the universe, all of them with their own planets yet to be discovered.